As climate change continues to pose significant challenges to our planet, innovative technologies are emerging as critical tools in our efforts to mitigate its effects. Satellite tracking and the Internet of Things (IoT) are at the forefront of this technological revolution, providing invaluable data and insights across various domains.
From monitoring endangered species and tracking glacial retreat to combating illegal fishing and preserving forest health, these technologies are playing a vital role in understanding and addressing climate change. This blog post explores five key ways in which satellite tracking and IoT are transforming the fight against climate change.
1. Tracking Endangered Species
While estimates vary, scientists agree that extinction rates are far higher than the natural rate, due to loss of habitat, climate change and poaching. A 2019 United Nations report puts the figure at 30 to 50 percent of all species going extinct by 2050. This loss of biodiversity threatens ecosystems that support all life – and there are further negative implications for medicine, agriculture and recreation.
Technology has a vital role to play in arresting this decline. Animal tracking through collars and tags helps in several ways: firstly, the data captured can help scientists prioritize habitat conservation, and justify seasonal closures of sensitive areas to the public.
Secondly, it helps us understand the impact of climate change, and both natural and human-driven disasters on wildlife, such as how sperm whales were affected by the Deepwater Horizon oil spill.
Finally, animal tracking can prevent poaching, both by helping to predict endangered species’ movements, and in turn, the hunters trying to evade detection. In this instance, tracking collars are often also combined with intelligent camera traps, giving security forces more information on the threat, so they can respond appropriately.
Satellite connectivity is essential for tracking animals traveling outside of cellular coverage. Modems such as the Iridium 9603N are increasingly small and lightweight, and can be built into the tracking collars for mammals starting at 15 Kg body weight.
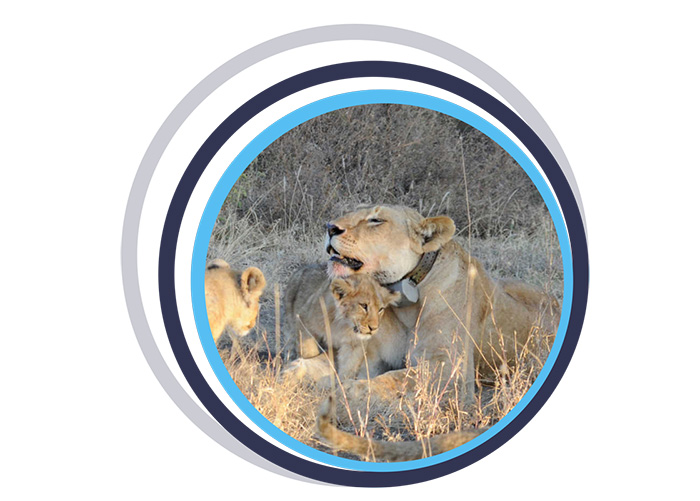
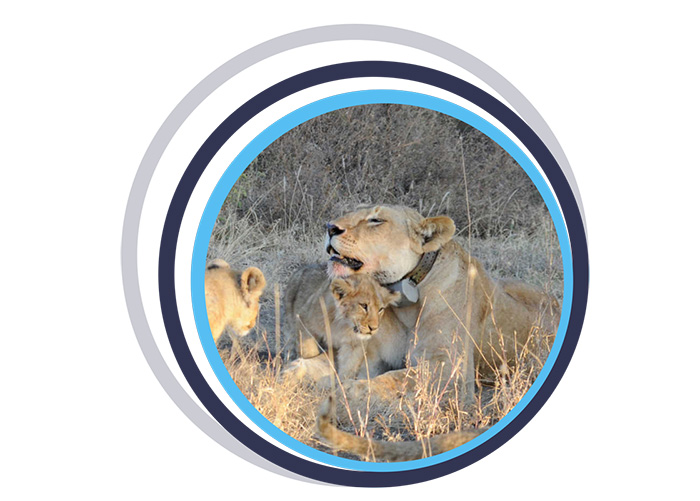
Contact us
2. Monitoring Glacial Retreat
Glaciers are shrinking rapidly, with profound impacts on local hydrology, rising global sea levels, and the acceleration of natural hazards such as the creation of icebergs. However, the processes that go into glacial retreat are not well understood, leaving gaps in models designed to predict future impact.
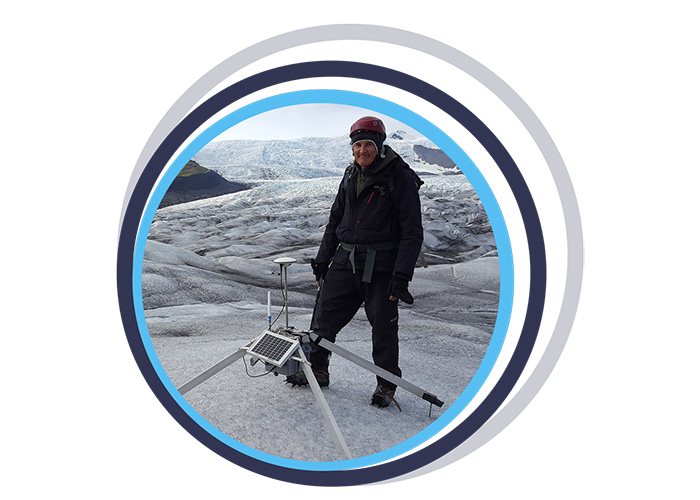
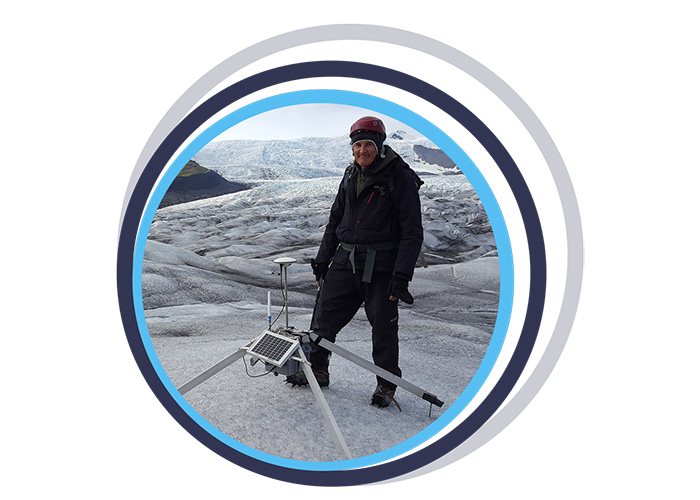
Environmental scientists are working hard to plug these gaps, not least the team at the University of Southampton, who build and deploy Subglacial Probes and Ice Trackers. The Ice Tracker is a web-connected RTK GNSS-solution which measures glacier change and flow. It utilizes the RockBLOCK 9602 to transmit its data reliably and cost-effectively, with no dependency on terrestrial network availability.
After rigorous testing in Iceland, the goal is to roll out this technology around the world to see how different glaciers respond to global warming, and thus create more accurate models for predicting their impact.
Contact us
Similarly, scientists from the Water and Ice Research Laboratory at Carleton University have developed a low-cost, satellite-enabled device to track icebergs. In the Arctic, as the sea ice retreats, more large icebergs are being calved; at the same time, shipping and fishing vessel traffic in the area has increased by 111% and 41% respectively.
Despite advances in monitoring, ships do still collide with icebergs; in the northern hemisphere, from 1980 to 2005, there were 57 incidents involving icebergs. With the addition of more icebergs and more ships, this risk has exponentially increased.
Tracking – and therefore being able to better predict the behavior of – icebergs mitigates the risks to marine vessels, and also supports scientific research into the effects of iceberg melt on ocean infrastructure and marine life.
The Cryologger is a data recording and telemetry platform that has been ruggedized so it can operate at Arctic temperatures. It utilizes a GNSS receiver, accelerometer, magnetometer and a RockBLOCK 9603 to transmit the data packets.
Data retrieved has already contributed to a database of iceberg tracking beacon tracks, providing insight into drift characteristics and distribution.
3. Combating Overfishing
According to Fishforward.eu, 29% of the world’s fish stocks are overfished; and a further 12-28% of the fishing world-wide is constituted by illegal and unregulated fishing. This is driven by demand, with each individual eating around twice as much fish as was consumed 50 years ago.
Right now, it’s other marine life that suffers the impact of overfishing. 70% of specific shark populations have been wiped out, and more than one-third of sharks, rays and skates are threatened with extinction.
And if nothing changes, in a few years time – some researchers predict as soon as 2048 – the world’s oceans will be virtually empty, leaving billions of people without their key source of protein, and millions of people missing their livelihood.
There are several solutions to this problem, as outlined by the Marine Stewardship Council; among them robust and enforced regulations preventing overfishing. The means of monitoring compliance is a Vessel Monitoring System, or VMS.
The device used to capture the telemetry on a vessel’s present and historical location, fuel used, catch size etc. needs to be tamper-proof and withstand the harsh marine environment. It needs to be reliable, accurate and have no connectivity ‘dead areas’.
VMS specialists Dualog (trading as Fangstr) and Pivotel chose the RockFLEET for their transmissions; it can use cellular when within range of a terrestrial network, and switches to the globally available Iridium satellite constellation when cellular is not available.
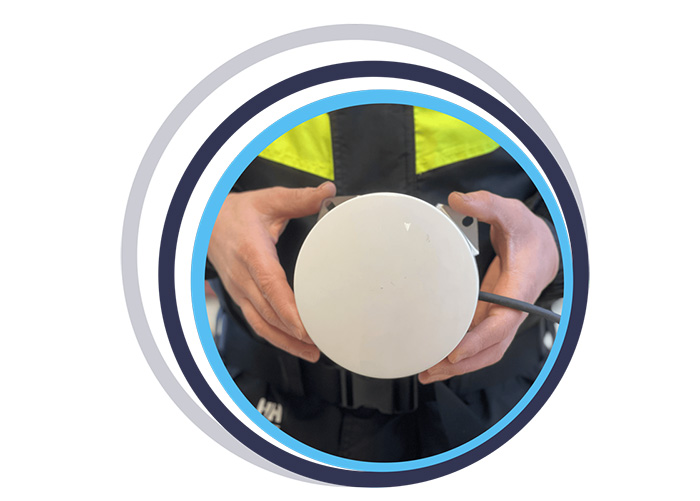
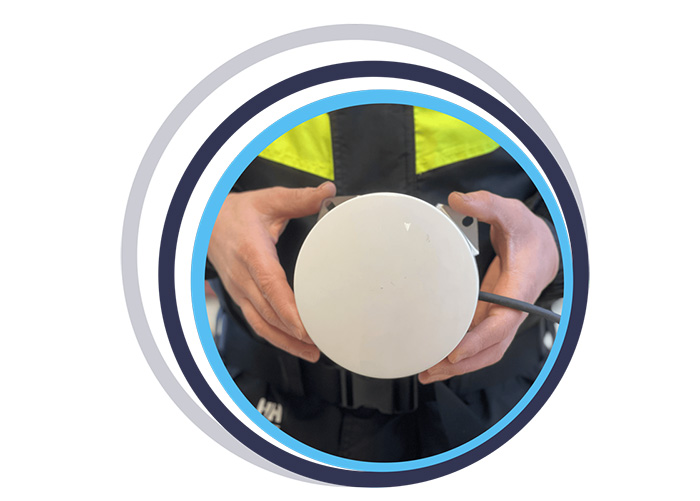
Contact us
4. Preventing Deforestation
Forests are both affected by climate change, and a key defense against it. Forests capture and store carbon, but when forests are cleared, burned or degraded, they release that carbon back into the atmosphere as carbon dioxide, which contributes to climate change.
Deforestation – the clearing of forests, usually to plant crops in its place – contributes 12 to 20 percent of global greenhouse gas emissions. Degraded forests also contribute; a degraded forest may emit more carbon than it captures, becoming a carbon source rather than a carbon sink. Degradation typically occurs when illegal logging operations take place; loggers bulldoze their way in, extract high value trees, and drag them out, leaving behind roads, clearings and ravaged undergrowth.
The Rainforest Foundation UK is supporting national and local authorities by providing an early warning system for illegal logging activities. They enlist the help of the indigenous people whose way of life is also being threatened by deforestation; when they see signs of illegal logging, mining, or oil spills, they use the free ‘ForestLink’ system to send an alert to the authorities.
When cellular coverage isn’t available, the ForestLink system switches to the global Iridium satellite constellation, allowing the monitors’ smartphones to exchange data anywhere with a clear view of the sky. Rainforest Foundation chose the RockBLOCK Plus for its ruggedized exterior and economical and reliable transmissions.
Contact us
5. Measuring Ocean Currents to Understand Global Climate Patterns
Our oceans absorb most of the sun’s heat, and then ocean currents distribute that heat around the globe. NOAA describes this as a conveyor belt, moving warm water and rain to the polar regions. There the water cools and sinks, which has the effect of pushing cold water towards the equator, helping to moderate temperatures. Without them, temperatures would be far more extreme – very hot at the equator, very cold at the poles – and much less of Earth’s land would be habitable.
Climate change is believed to be affecting ocean currents; the currents are not only warmer, but also 15 percent faster (measured between 1990 and 2013). This is damaging marine life and speeding up the melting of the sea ice at the poles, leading to global sea level rises. It’s also likely to disrupt the conveyor belt; if the water reaching the poles is too warm, it won’t sink. This could have the effect of slowing down or even stopping ocean currents in some places, notably the Gulf Stream. Western Europe would feel very different without the effect of this warming current.
High sea surface temperature (SST) is an important parameter in predictive weather and climate modeling. To capture this data, fixed and drifting data buoys are deployed all over the world to take measurements of surface and subsurface water temperature, atmospheric pressure, winds, salinity and wave patterns. And for the drifting data buoys, their historical location data allows scientists to profile ocean currents.
Because many of these data buoys are outside of cellular coverage, satellite connectivity is essential to transmit their data. Ground Control works with a number of data buoy companies, who need a satellite modem that’s reliable, robust, and delivers global coverage. RockBLOCK 9603 is a popular choice as it’s cost effective and has very low power consumption, allowing for the data buoys to drift for several years on battery power, or a small solar panel.
Some of Ground Control’s data buoy partners include Sofar Ocean, Maker Buoy, Akrocean, Running Tide and MTE Instruments.
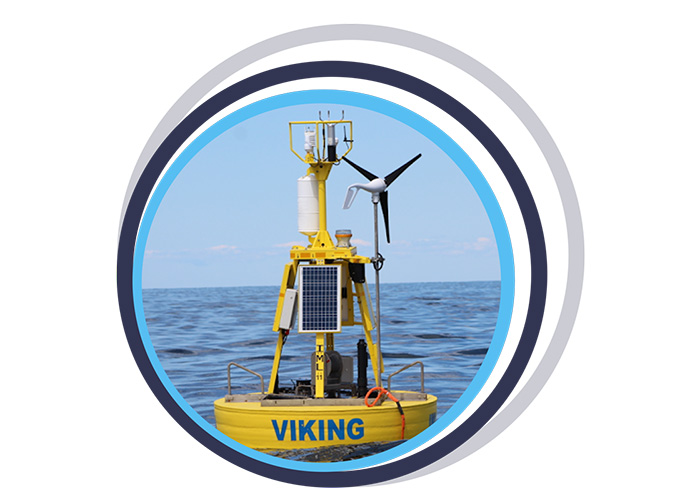
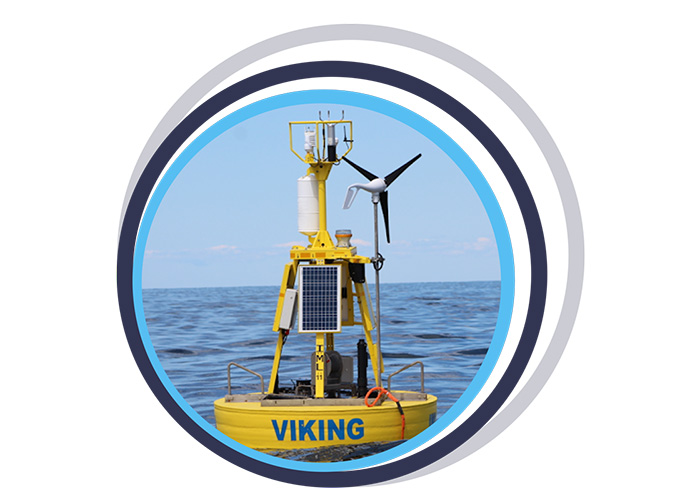
Contact us
In Summary
The integration of satellite tracking and IoT technologies offers powerful solutions to some of the most pressing environmental challenges we face today. By enabling real-time data collection and analysis, these technologies help scientists, conservationists, and policymakers make informed decisions to protect our planet.
As we continue to innovate and expand the applications of these tools, their role in combating climate change will only become more significant. Embracing and investing in these technologies is essential for creating a sustainable future for generations to come.
Can we Support You?
Based in the UK and USA, Ground Control designs and builds satellite-enabled tracking and IoT solutions. We have over 20 years’ experience, and work with leading satellite network operators to ensure all of our customers get the best combination of coverage, cost, data throughput and latency.
If you have an asset that’s moving in and out of cellular coverage, we ensure that you always stay connected. We work directly with end users like Digital Forest, and often indirectly through our partner network of companies building animal tracking collars and data buoys, for example.
Please email hello@groundcontrol.com or complete the form, and we’ll reply within one working day.